Last
blog we learned about the strange things happening to gas in dense environments, like the Virgo cluster. This blog, we are going to continue in that vein. But first, a refresher on why 13CO is interesting.
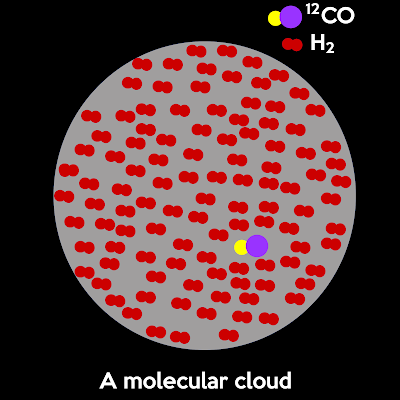 |
Cartoon version of a molecular cloud. Image credit: Katey Alatalo |
If we had eyes that could look at a molecular cloud, we would see a ton of molecular hydrogen, and a smattering of other elements. Namely, molecular gas is nearly all molecular hydrogen, with the second most abundant molecule being carbon monoxide (CO). In fact, for every 10,000 hydrogen molecules, there is 1 CO molecule. So why is it that we use CO when we study cold gas in galaxies, rather than molecular hydrogen? Because of their dipole moments. As shown in the figure, H2 has two symmetric atoms that make it up, meaning that if it rotates, it does not release a dipole electric charge, meaning that it has very weak emission. CO on the other hand, is made out of asymmetric atoms (the 12 nucleon-carbon and the 16-nucleon oxygen), so it creates a large dipole moment and thus very strong emission.
There are some caveats of course. First off, in the clouds, both the H2 and the CO are usually optically thick. That means that we are not counting every single emitting photon. This normally would be a problem, but it was found that in molecular clouds, the total luminosity in CO was related to the total mass of the clouds, something called
Larson's law. This means that in many cases, we are able to use the CO luminosity to trace the underlying mass of molecular gas. This might not be true in very low metallicity sources, which have not been enriched yet with carbon and oxygen, and this relation comes with a large set of uncertainties. One of the ways that we compensate for those uncertainties is by turning to optically
thin molecules. The easiest one to observe is also a species of CO, but this time it is 13CO (that is a 13-nucleon carbon and a 16-nucleon oxygen). This isotope is about 70x less abundant than 12CO, and is thought to be optically thin. This means that it should provide a more faithful accounting of the total molecular mass, because we are able to count every emitting molecule. This also means the line is intrinsically weaker than the 12CO line. And for the paper I am blogging, it was the 13CO that we set out to detect.
We turned to the 17 galaxies from the
ATLAS3D CARMA survey that we had simultaneously observed both the 12CO and the 13CO lines. The 13CO ratio can differ from source to source for a lot of reasons, so we were expecting a random scatter of sources. But the scatter was not random, not at all. Virgo early-type galaxies have higher 13CO/12CO ratios than field galaxies.
 |
The distribution of 12CO/13CO ratios in early-type galaxies (ETGs) that shows that Virgo has elevated ratios compared to field galaxies (those not in clusters). Adapted from Alatalo et al. 2015. |
What could this mean? There are plenty of ways we can do this, but here are a few that might also work in the context of Virgo galaxies having aligned molecular gas and equivalent detection rates to field early-type galaxies. First is
midplane pressure. In Virgo, the galaxies are subjected to extra pressure from the intracluster medium (the hot gas that fills the space between the galaxies in the Virgo cluster), which could increase optical depth of the gas in Virgo ETGs. This though would not impact the detection rates. Second is
enrichment. Different types and masses of stars create different carbon isotopes when they are undergoing fusion. This means that in galaxies that has gas that has been recycled over and over, the gas can become more enriched in one isotope than in another. Given the lack of primordial gas in Virgo galaxies, this could certainly have changed the abundance of 13C relative to 12C. Though again, is unable to explain the same detection rates. Finally, it could be
preferential stripping of low mass molecular clouds (coined survival of the densest). Ram pressure stripping can strip away atomic and unbound gas, but perhaps it does not stop there, and also is able to remove the bound molecular gas in the smaller molecular clouds, leaving only the largest. This would mean that the 13CO would also become close to being optically thick, and is similar to the ratio seen in the largest molecular clouds in the Milky Way. Or, it could be
none of the above, and we could be seeing a mass effect. The early-type galaxies in Virgo are the most massive, so this relation could simply say that massive galaxies retain their gas for longer.
The question of why Virgo galaxies appear to be special is not yet resolved. We don't know why the detection rates are the same (looking at more early-types in more clusters will help). We also don't know why the galaxies in Virgo seem to have more dense gas than their field galaxy counterparts. But high resolution observations on scales of the molecular clouds in Virgo (both spirals and ellipticals,
and in the outskirts and internally), perhaps we can start to distinguish between possible mechanisms.
The official published version can be found on
NASA ADS.
Get a PDF version made by me
here.